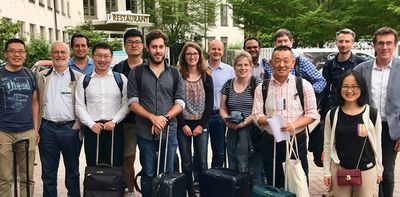
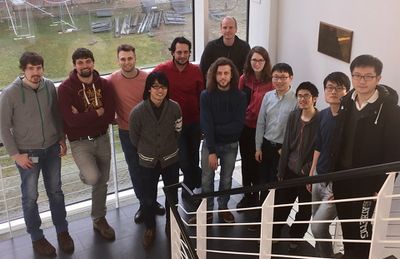
Group members
Name | Role |
---|---|
Rafal E. Dunin-Borkowski | Professor; Director of PGI-5 |
András Kovács | Permanent scientific staff |
Yoshie Murooka | Scientific staff |
Amir H. Tavabi | Scientific staff |
Thibaud Denneulin | Scientific staff |
Qianqian Lan | Scientific staff |
Janghyun Jo | Scientific staff |
Fengshan Zheng | Scientific staff |
Benjamin Zingsem | Scientific staff |
Peng-Han Lu | PhD student |
Teresa Wessels | PhD student |
Tanvi Bhatnagar | PhD student with JCNS? |
Giulio Pozzi | Visiting Professor |
Vincenzo Grillo | Visiting Scientist |
Former group members
Name | Role | Period |
---|---|---|
Dongsheng Song | Scientific staff | 2018 - 2020 |
Elisabeth Josten | Scientific staff | |
Vadim Migunov | Scientific staff | 2014 - 2020 |
Jan Caron | Scientific staff | |
Patrick Diehle | PhD student | |
Trevor Almeida | Visiting Scientist |
Research fields
Electric field mapping
Projected electrostatic potential and charge density mapping
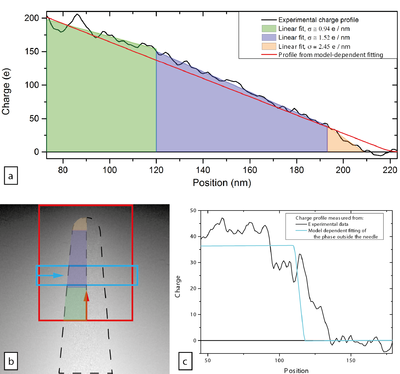
The distribution of electric field around a needle shaped sample is crucial information for design of novel field emitting devices and atom probe tomography (APT).
We have used off-axis electron holography combined with in situ electrical biasing to measure distribution of projected electrostatic potential which is encoded in the electron phase shift. [1] The analyses of the data can be performed using both model-dependent and model-independent approach. The first one utilises fitting of projected electrostatic potential to analytical model for line of charges which describes potential distribution around charged rotational ellipsoid. [2] The model-independent approach relies on calculation of projected charge density from the phase maps by using either 2D Laplacian operator [3] or by loop integration[4]. Both approaches can be combined: model-independent one can be used to refine the model and with assumption of symmetry, refined model can be used for calculations of 3D potential distribution.
Performing full three-dimensional reconstruction of potential or charge density would take this work forward.
Further reading: [5]
Further contact: Vadim Migunov, Fengshan Zheng
Three dimensional mapping of electrostatic potential, electric field and charge density
- Analytic model-dependent approach[6]
- Model-independent approach[7]
- Model-based iterative reconstruction[8]
Further contact: Fengshan Zheng, Vadim Migunov
Magnetic field mapping
Magnetic vector field tomography
Shaping electron beams
In situ electron microscopy
Methods, instrumentation and software
Methods
- Lorentz TEM
- Off-axis electron holography
- Large field of view holography
- Electron Magnetic Circular Dichroism
- In situ electrical biasing
Instrumentation
- K2-IS camera on Holo microscope
- DENS Single tilt heating holder
- DENS Double tilt cooling heating biasing holder
- Nanofactory STM-TEM holder
Software
- HyperSpy - multidimensional data analyses and hologram reconstruction
- Pyramid - 2D and 3D magnetisation and electric charge reconstruction from electron phase images
- ASTRA-toolbox - 3D tomography reconstruction
- LiberTEM - pixelated STEM data analyses
- Gatan Scripting
- MR Ruska (Microscopy Robotization in Ernst Ruska-Centre) - a set of GMS3 scripts for automation of microscopy routines.
- Holoworks - a plugin in GMS3 for hologram reconstruction
Data analyses
Pages
- Holo microscope status
- Holo group meetings
- Workflows and guides
- Knowledge Base
- Möllenstedt (IFF588) server
References
- ↑ http://dx.doi.org/10.1063/1.4916609 Migunov, V., London, A., Farle, M. & Dunin-Borkowski, R. E. Model-independent measurement of the charge density distribution along an Fe atom probe needle using off-axis electron holography without mean inner potential effects. Journal of Applied Physics 117, 134301 (2015).
- ↑ http://doi.org/10.1016/0304-3991(92)90039-M Matteucci, G., Missiroli, G. F., Muccini, M. & Pozzi, G. Electron holography in the study of the electrostatic fields: the case of charged microtips. Ultramicroscopy 45, 77–83 (1992).
- ↑ http://dx.doi.org/10.1063/1.3598468 Beleggia, M., Kasama, T., Dunin-Borkowski, R. E., Hofmann, S. & Pozzi, G. Direct measurement of the charge distribution along a biased carbon nanotube bundle using electron holography. Applied Physics Letters 98, 243101 (2011).
- ↑ http://dx.doi.org/10.1103/PhysRevLett.111.025501 Gatel, C., Lubk, A., Pozzi, G., Snoeck, E. & Hÿtch, M. Counting Elementary Charges on Nanoparticles by Electron Holography. Phys. Rev. Lett. 111, 25501 (2013).
- ↑ http://dx.doi.org/10.1063/1.4916609 Migunov, V., London, A., Farle, M. & Dunin-Borkowski, R. E. Model-independent measurement of the charge density distribution along an Fe atom probe needle using off-axis electron holography without mean inner potential effects. Journal of Applied Physics 117, 134301 (2015).
- ↑ http://dx.doi.org/10.1063/1.4916609 Migunov, V., London, A., Farle, M. & Dunin-Borkowski, R. E. Model-independent measurement of the charge density distribution along an Fe atom probe needle using off-axis electron holography without mean inner potential effects. Journal of Applied Physics 117, 134301 (2015).
- ↑ http://dx.doi.org/10.1063/1.3598468 Beleggia, M., Kasama, T., Dunin-Borkowski, R. E., Hofmann, S. & Pozzi, G. Direct measurement of the charge distribution along a biased carbon nanotube bundle using electron holography. Applied Physics Letters 98, 243101 (2011).
- ↑ https://www.sciencedirect.com/science/article/pii/S0368204818302032 Zheng, F., Caron, J., Migunov, V., Beleggia, M., Pozzi, G. & Dunin-Borkowski, R. E. Measurement of charge density in nanoscale materials using off-axis electron holography. Journal of Electron Spectroscopy and Related Phenomena, in press.